Inductively Coupled Plasma (ICP) - MS
Greet de Loos-Vollebregt, Ghent University, The NetherlandsAbstract
ICP-MS is widely used for elemental detection in combination with various methods for separation, including GC, HPLC, CE and ion chromatography. Detection limits are extremely low, in the nanogram per liter range.
Numerous applications of speciation analysis have been reported, mainly in the field of biological systems, food analysis and environmental chemistry.
The mass spectrum presents the single charged nuclides of elements present in the inductively coupled plasma discharge. The signal intensities correspond with the element concentrations in the sample solution.
LevelBasic
Analytical characteristics and applications of ICP-MS in speciation analysis
ICP-MS is widely used for elemental detection in combination with various methods for separation, including GC, HPLC, CE and ion chromatography. Detection limits are extremely low, in the nanogram per liter range.
Numerous applications of speciation analysis have been reported, mainly in the field of biological systems, food analysis and environmental chemistry. References to publications and information on species certified reference materials are found at the website of the European Virtual Institute for Speciation Analysis (EVISA): http://www.speciation.net/
Inductively coupled plasma: for explanation on ICP and sample introduction see the corresponding paragraph on ICP-OES.
Principle
Upon heating to 5000 K in the plasma most elements are present as single-charged ions (+1) which can be transferred to a mass spectrometer. The inorganic mass spectrum shows the intensities of nuclides and a few poly atomic species in the m/z range up to about 300. The intensities correspond to the concentrations of the elements in the sample solution.
Molecular information is lost in atomic spectroscopy detection methods because the sample must be heated to a temperature that is high enough to generate atoms and/or ions of the elements of interest. However, the combination of both organic and inorganic mass spectrometry coupled to separation techniques can offer full analytical characterization of all species.
Generation of ions in the plasma
The degree of ionization, α, varies from 100% for alkali and alkali earth elements to about 30% for elements with a high ionization energy (e.g. As and Se).
where [M] is the atom density and [M+] is the ion density. The Saha equation shows that the ionization equilibrium depends on the ionization energy Vion and ionization temperature Tion
where ne is the electron density, me is the electron mass, Te is the electron temperature, h is the Planck constant, Za and Zi the partition function for the atom and ion, respectively and k is the Boltzmann constant. The ICP is an excellent source for the formation of single-charged ions
Element | Ionization Energy (Ev) | Degree of ionization (%) |
Na | 5.14 | 100 |
Ca | 6.11 | 99 |
Pb | 7.42 | 97 |
Se | 9.75 | 33 |
As | 9.79 | 52 |
Ar | 15.76 | 0.04 |
Instrumentation
Inductively coupled plasma: for explanation on ICP and sample introduction see the corresponding paragraph on ICP-OES.
ICP-MS instrument: The ions formed in the plasma are extracted via a conical water-cooled sampler into the first vacuum stage where a pressure of ~100 Pa is maintained and then pass through the skimmer placed a few cm behind the sampler. Ion lenses focus the ion beam in the high vacuum (~10-3 Pa) provided by a turbomolecular pump. The detector measures the ion intensities.
ICP-MS Instrument
The ICP-MS instrument consists of the following components:
-
Torch
-
Power supply and load coil
-
Sample introduction system (nebulizer and spray chamber)
-
Mass spectrometer (quadrupole, sector field, time of flight or multicollector)
-
Detection system (electron multiplier, Faraday cup)
More information on the inductively coupled plasma is found in the section on ICP-OES.
The mass spectrometers used in ICP-MS are basically the same as used in GC/MS and LC/MS.
Isotopes
Isotopes: Nuclides having the same atomic number but different mass number are called isotopic nuclides or isotopes. Isotopes have the same number of protons and electrons, but differ in the number of neutrons present in the nucleus. The natural abundance of a stable isotope is the percentage of occurrence (between parentheses).
O | 16O (99.76%), 17O (0.048%), 18O (0.20%) |
Cl | 35Cl (75.77%), 37Cl (24.23%) |
Ar | 36Ar (0.337%), 38Ar (0.063%), 40Ar (99.600%) |
As | 75As (100%) |
Se | 74Se (0.9%), 76Se (9.2%), 77Se (7.6%), 78Se (23.7%), 80Se (49.8%), 82Se (8.8%) |
Sn | 112Sn (0.97%), 114Sn (0.65%), 115Sn (0.36%), 116Sn (14.53%), 117Sn (7.68%), 118Sn (24.22%), 119Sn (8.58%), 120Sn (32.59%), 122Sn (4.63%), 124Sn (5.79%) |
Hg | 196Hg (0.15%), 198Hg (10.1%), 199Hg (17.0%), 200Hg (23.1%), 201Hg (13.2%), 202Hg (29.65%), 204Hg (6.85%) |
Pb | 204Pb (1.4%), 206Pb (24.1%), 207Pb (22.1%), 208Pb (52.4%) |
Mass spectrum for several elements
Mass spectrum for several elementsWe prefer to measure the intensities of selected isotopes with high(est) abundances unless interferences occur at a specific m/z.
Mass spectrum for several elements
Interferences
-
Isobaric interferences are caused by overlap between signals from nuclides having the same mass number but different atomic numbers (isobaric nuclides or isobars). The relative isotopic abundance of the naturally occurring elements is well known and therefore potential isobaric interferences are predictable. They can often be corrected by mathematical correction equations, measuring the interfering nuclide at another mass which is free of interference.
-
Spectral interferences. Polyatomic or molecular spectral interferences can be generated by either argon-, solvent or sample-based ionic species, e.g. the interference of 40Ar16O+ on 56Fe+, 40Ar35Cl+ on 75As+ or 40Ar40Ar+ on 80Se+. Polyatomic spectral interferences can be minimized or eliminated by the application of correction equations, cool plasma technology or matrix separation.
-
Oxides, hydroxides, doubly charged species. Oxides and hydroxides may occur at 16 or 17 mass units higher than the analyte mass and create spectral overlap problems. Doubly charged ions may cause interferences at half of their mass. The formation of oxides and double-charged ions is related to the ionization conditions in the plasma and can be minimized by appropriate optimization of the experimental conditions, e.g. nebulizer gas flow and RF power. Such interferences are typically observed for rare-earth and refractory elements (e.g. CaO+, BaO+, CeO+, CaOH+, POH+, BrH+, Ba2+).
-
Matrix interferences. The signal may be influenced by the sample matrix, e.g. due to changes in droplet formation or transport efficiency or changes in the plasma ionization conditions. Such problems can usually be solved by internal standardization.
Resolution
Resolving power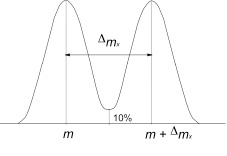
For many applications it is sufficient to measure m/z values without decimal positions, which can easily be achieved with a quadrupole mass spectrometer. The resolution would then increase from 7 for 7Li+ up to 208 for 208Pb+. This would not be enough to separate the peaks of 56Fe (m/z = 55.934939 and 40Ar16O (m/z = 55.957298) or 75As (m/z = 74.921594 and 40Ar35Cl (m/z = 74.931236). Better resolution can be achieved with time of flight and sector field mass spectrometers. For further information on mass spectrometers see Chromedia sections on GC/MS and LC/MS.
Exact mass | Polyatomic ion | Resolution required for separation |
16O (m/z = 15.994915) | ||
35Cl (m/z = 34.968852) | ||
40Ar (m/z = 39.962384) |
|
|
56Fe (m/z = 55.934939)
| 40Ar16O (m/z = 55.957299) | 2500 |
75As (m/z = 74.921594) | 40Ar35Cl (m/z = 74.931236) | 7800 |
Separation of As from ArClSeparation of 75As+ from 40Ar35Cl+ using the high resolving power (R = 10 000) of a sector field ICP-MS instrument (Thermo Finnigan).